Bovine lysosomal α –mannosidase (PDB ID:1O7D) from Bos taurus
Created by: Chih-Huan Lu
Bovine lysosomal α -mannosidase (1O7D) from Bos Taurus is a glycoside hydrolase that’s specified to breakdown α -mannosidic linkages (1). The enzyme resides in lysosome and functions in breaking down mannose-glycans (2) made mostly from catabolism of glycproteins, especially N-linked saccharides. Bovine lysosomal α –mannosidase (bLAM) is a member of the Glycoside Hydrolase Family 38, which contains class II α –mannosidases (1). These GH38 mannosidases reside in different regions of the cell and have different specificities of their cleavages. Mammals have three types of GH38 α –mannosidases: Golgi α –mannosidases, lysosomal α –mannosidases, and cytosolic α –mannosidases (1,3). Golgi α –mannosidases have 2 specificities for α–1,6 and α–1,3 linkages and lysosomal α –mannosidases have ranges of specificities from narrow (α–1,6 only) to broad (α–1,6, α–1,2, α–1,3) (3,4). bLAM is one of the α –mannosidases that has broad specificity and cleaves all known α linkages. (1,4)
Decrease of bLAM activities result in α –mannosidosis in cattles, same for humans, cats, and other mammals with loss of LAM functions. α –mannosidosis is an autosomal recessive genetic disorder arises from mutations in MAN2B1 gene (5). The deficiency of α –mannosidase activity leads to lysosomal accumulation of mannose-rich oligosaccharides in cells and disrupt normal cell functions (5). α –mannosidosis in human causes hearing impairment, skeletal and neurological abnormality, recurring infections and psychiatric problems, and mental retardation (1,5); it leads to early fatalities in severe (type I) α –mannosidosis. Although there are no cure, ongoing research with human stem cell transplantation and recombinant emzyme replacement therapy offer some treatment to improve the conditions (5). Since bLAM is very similar to human LAM both structurally and functionally, one of the reasons it’s studied is to serve as a model to human enzyme and hopefully shed light on α –mannosidosis research.
The cleaving of α–linkages of α –mannosidase is through retaining Koshland double-displacement mechanism (3). The 2-step catalysis involves two carboxylated groups on the enzyme, usually glutamate or aspartate, one functions as a nucleophile and the other functions as a acid/base group (1,6). First the nucleophile attacks the anomeric carbon to form the covalent bond while the broken off α–linkage glycosidic oxygen got protonated by the acid/base group, creating the glycosyl enzyme intermediate (6). Next, acting as a base, the deprotonated acid/base group activates a water molecule for it to hydrolyze the intermediate to form product (6). The catalytic nucleophile is identified to be
D196 (1). The scheme of the mechanism(6):
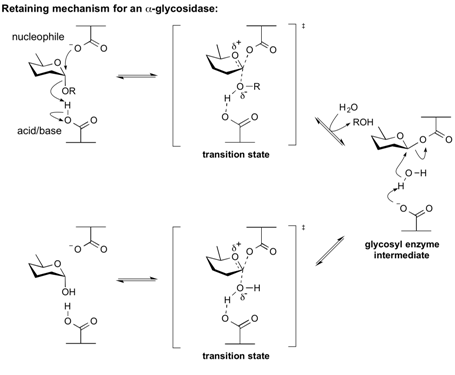
Another reason that bLAM is studied is that it’s activation in lysosome might be a new mechanism compared to other activation methods. The activation is not likely due to protein interactions because bLAM transport is compartmentalized and that the protein will be transported further after dropping off bLAM in lysosome (1). The activation is also not due to timed proteolysis as pre- and post-proteolytic bLAM show similar activities (1). The postulated activation mechanism is that the domains move and rearrange to active form as ionic interactions weakened in low pH (1). This is plausible as that bLAM has three interdomain ionic networks composed of many acidic amino acids; these amino acids will be protonated in lysosome’s low pH and the interactions disrupted (1). The three ionic bond network:
peptide loop between D domain β sheets 16 and 17, interactions between C and D domain interface, and residues from A,B,D,E chains that stabilize the active sites (1). There are also some other charged structures that can act as flexible linkers between domains that allow large domain movements in bLAM such as
α helix D1, β sheets D18 ,E1 and the
three helices (B2,C1,C2) (1).
bLAM is a globular protein composed of
five chains A-E and is folded into four different domains (1). 5 chains have different proportion of
secondary structures: chain A contains 38% α-helices and 14% β-sheets; chain B contains 42% α-helices and 11% β-sheets; chain C contains 29% α-helices and 31% β-sheets; chain D contains 8% α-helices and 42% β-sheets; and chain E contains 2% α-helices and 37% β-sheets (7). A and B chains form the N-terminal active site containing domain with both α helixes and β sheets. C, D, and E chains form the other three β sheets domains accordingly with a
three helices bundle connecting B and C chain (1). The
active site in N-termial domain is formed by 7 parallel distorted β-barrel core surrounded by 10 connecting α-helices (1). The domain is stabilized by two disulfide bridges:
C268 and C273 both on chain A stabilize a loop on the dimer interface, and
A:C55(A chain C55) with B:C358(B chain C358) bridge connects the N terminus to the domain (1).
Although only showing the monomer here, bLAM actually forms dimers by interactions between two active site containing domains, forming a central depression area (1). The active site of bLAM is on the side of the central cleft, completed by a loop in chain C and two loops in chain D (1). The active site of bLAM is coordinated to
Zn2+ and
Tris molecule that aid the enzyme in substrate binding and active site functioning (1). The zinc ion is coordinated by 2 aspartic acid residues and 2 histidine residues: A:D74, A:D196, A:H72, and C:H446. Zinc ion is critical for the enzyme function as that one of the residue that it’s coordinated to, A:D196, is the catalytic nucleophile. Tris molecule (C4H12NO3) is also bound in the active site with its three oxygen hydrogen-bonded to D:Y660, A:R220, and A:D74 (1). A:R220, which is also hydrogen bonded to nucleophile A:D196, is an important residue that participates in substrate binding in the active site (1). There are three possible candidates for general acid/base residues that are close enough to A:D196 to perform the catalytic function: A:D319, A:D74, and C:D447 (1). A:D319 might be the most probable one since its analogue in the closely related dorsophila golgi α-mannosidase II carries out the acid/base function (1). But it turns out that A:D74 is coordinated with Zn ion and C:D447 is hydrogen bonded to C:H445, which both raised their pKa and make them better acid/base residues (1). The exact residue that contributes to the catalytic function of the enzyme remains unidentified.
The analysis of mutations that cause α-mannosidosis shows how individual residue is important to the function and stability of the enzyme. In active site, A:R220H mutation causes severe α-mannosidosis in cattles with neurological symptoms and early death (1). H220 can still hydrogen bond to D196 but can no longer hydrogen bond to
Tris and substrate, the mutation thus causes diminished substrate binding and destabilized catalytic neucleophile (1). Another mutation A:F320L results in severe α-mannosidosis in cattles (1). The aromatic ring of
A:F320 stacks against A:Y84 and anchors the possible acid/base A:D319 next to it; the mutation disrupts the stacking interaction and results in unstable catalytic components and unstable protein structure (1). Other mutations in active site or other domains that disrupt the catalytic function of the enzyme or the protein stability can also lead to α-mannosidosis.
DALI server uses a program to compare protein’s 3D structure and tertiary structures to look for similarity in the structures. The results are given in z score, the greater the score the more similar the structures. The program indicates that
Golgi α –mannosidase II (1HTY) is the most similar
structural homolog of bLAM with a z score of 28.0 (8). Golgi α –mannosidase II is an enzyme in golgi that process N-glycan, which is interested in its relationship with complex carbohydrate structures on the cancer cell surface and potentials for cancer therapy (9). The result makes sense as that the Golgi α –mannosidase II and Lysosomal α –mannosidase are in the same hydrolase family and carry out similar catalytic functions. Searching for primary sequence similarity, the BLAST result gives Golgi α –mannosidase II of Dorsophila with e value in the order of e
-80 (10). An e value of 0 indicates exact primary sequence match, and e
-80 is a very small number that indicates large amount of homogeneity of the two protein primary sequences. Along in the BLAST result are many other Lysosomal α –mannosidase from different species with extremely low e values. Most similar homolog of non-lysosomal α-mannosidase is alpha-mannosidase from Capra hircus also with e value of 0 (10). These data suggest that although not much, there are still differences between Golgi α –mannosidase II and Lysosomal α –mannosidase. Using the ExPASy program, a bioinformatic tool that calculate physical properties of the protein based on primary sequence, bLAM has molecular weight of 107.8557 Kd and a theoretical isoelectric point of 8.99 (11).